Translate this page into:
Impact of Pneumatic Transport System on Preanalytical Phase Affecting Clinical Biochemistry Results
Address for correspondence: Ravi Shekhar, MBBS, MD, Biochemistry Department, Indira Gandhi Institute of Medical Sciences, Sheikhpura, Patna, Bihar 800014, India (e-mail: ravishekhar1974@yahoo.com).
This article was originally published by Thieme Medical and Scientific Publishers Pvt. Ltd. and was migrated to Scientific Scholar after the change of Publisher.
Abstract
Introduction
PTS (pneumatic transport system) is extensively being used in modern hospitals for rapid transportation of blood samples and other specimens. However, it has a potential impact on blood components, which should be investigated and nullified accordingly. This study was part of a correction program aimed at reducing hemolysis. It was done by comparing paired samples transported manually and by PTS.
Materials and Methods
This study was initiated to monitor the impact of PTS on hemolysis of clinical biochemistry blood samples. It was performed in two phases—before and after the corrective action taken. Phase I: done after PTS installation but before the corrective action was taken. Duplicate samples from 100 healthy individuals were collected, one set transported by PTS and the other by human carriers. Both sets were assessed for 25 biochemistry analytes, hemolysis index (HI), and acceleration profiles using a data logger. Corrective measures were then taken, followed by phase II of the study. In phase II, the sample size and study design remained the same as phase I. All the test results of PTS and hand-carried samples were statistically analyzed for any significant difference.
Result
In phase I, all the hemolysis-manifesting parameters, LDH (lactate dehydrogenase), potassium, AST (aspartate transaminase), and phosphorus, were raised in PTS samples as compared with the manual samples. Their differences were significant as the p-values were 0.001, 0.000, 0.025, and 0.047, respectively. The differences for LDH and potassium were clinically significant as well. HI (9%) and peak acceleration (15.7 g) were high in PTS samples.
In phase II, no statistically significant difference between paired samples was found for all biochemistry parameters except for a few which were clinically nonsignificant. For PTS samples, HI was 2.5% and the peak acceleration was 11.2 g, whereas for manual samples, HI was 2%.
Conclusion
Evidence of hemolysis was found in PTS samples as compared with handheld samples, which was resolved after several corrective actions were taken. Thereafter, PTS became reliable for sample delivery in a routine biochemistry laboratory. Hence, each hospital should scrutinize their PTS for its effects on sample integrity to get rid of PTS-induced preanalytical errors.
Keywords
Pneumatic transport system
turn-around time
hemolysis
preanalytical errors
potassium
lactate dehydrogenase
Introduction
The turn-around time (TAT) is one of the most important quality indicators for the quality management system of a clinical laboratory.[1] It can be reduced by various measures, the most vital being minimization of transportation time of the specimen and samples to the laboratory.[2] To achieve this target, hospitals widely use the pneumatic transport system (PTS) for rapid and effective transport of blood samples.[3-5] PTS is a network of hollow tubes that allow users to send multiple carriers or capsules containing samples to a freely chosen destination. These destinations are supplied with stations that help to send or receive the carrier. In hospital settings, these carriers and stations are used for transporting blood and tissue specimen. It can also be exploited for sending medicines (cytotoxic or cytostatic), small goods, consumables, or for their inter-departmental exchange.[6]
With the installation and effective implementation of PTS, the mean TAT has reduced by 16 to 24%. And when PTS is associated with computerized physician order management, the TAT is even further reduced by 33 to 44%.[3,7,8] Various other advantages of PTS are reduced personnel requirement, cost efficiency, hygiene, round-the-clock service, and ease of use. PTS enables widespread access to central laboratory equipment and competes with the convenience of point-of-care testing stations.[9] However, several studies described that PTS significantly affects the blood sample integrity.[5,10,11] The factors implicated in the overall sample quality are shearing stress applied on blood cells due to acceleration and deceleration, high transportation speed, sudden change in the direction of carriers, movement and vibration of blood sample in the tubes, and lack of cushioning.[12] These dynamic elements increase the interaction both with the neighboring cells and with the wall of the samples' tube. It may culminate in increased hemolysis, which manifests as raised serum potassium, lactate dehydrogenase (LDH), aspartate transaminase (AST), etc. It may also contribute to platelet exhaustion.[13] All these studies provided evidence indicating that PTS may affect the samples in the preanalytical phase which may influence the final reporting.
The preanalytical phase in the laboratory reporting consists of ordering the test, proper specimen collection, specimen processing, transportation, and storage.[14-16] It is the largest contributor to errors in the laboratory-reporting process. According to several studies, this phase accounts for 60 to 70% of all errors occurring in the diagnostic laboratory.[17] With the advent of the efficient random-access analyzer, the error in the analytical phase is reduced to its minimal. The postanalytical phase also does not contribute much to the total error in the reporting. So, to diminish preanalytical errors imposed due to unwanted effects of PTS, it should be scrutinized on a regular basis. Each PTS is unique in terms of specification and configuration and it is assembled according to the explicit need of any health care system. So, Sodi et al emphasized that the user should evaluate the effects of their specific PTS system on the blood samples and take corrective action accordingly.[18] There are many published studies indicating PTS posing different kinds of effects on samples in different laboratories.
This study aims to monitor the effects of the PTS system installed in our hospital on blood sample integrity leading to hemolysis. Remedial measures would be taken if any major shortcomings were found. Samples would be again assessed after the curative measures were taken to ensure that PTS is no longer affecting the blood components.
Materials and Methods
Background
Ever since the installation of PTS (May 2019) in the central collection area, complaints have been received from clinicians of our institute regarding reporting of potassium, LDH, etc. The frequency of sample rejection due to hemolysis has also increased. So, this study was planned to examine the influence of PTS on the hemolysis of biochemistry samples. On complaints of hemolysis by the biochemistry department, the engineers of the PTS service provider (Kopran Ltd.) took some corrective actions to combat the problem. They employed cushioning in the carrier and below the station from where carriers are received, reprogrammed the path so that the shortest possible path could be traversed by the carrier, and decreased the blower pressure applied by the blower on the carrier. The earlier blower pressure was ± 160 mbar (± 10 mbar); same strength being used for suction and forward pressure, but later on there was 35% reduction in it on complaints of hemolysis. So that the present pressure is ± 105 mbar (± 7 mbar). Hence the acceleration decreased from its previous level and the time taken by the carrier to reach the Clinical Biochemistry Laboratory (CBL) increased from a mean of 61 (± 10) seconds to 106 (± 7) seconds. The velocity remained varied between 3 and 6 m/s during the entire path.
Study Design and Statistical Analysis
This is an analytical study, performed in two phases—phase I: before the corrective action, and phase II: after the corrective action taken to verify the effectiveness of the corrective measures taken. In phase I, samples were collected to scrutinize the effects of PTS on blood cells' integrity, before the corrective measures were taken. For this 4 (2 containing clot activator and 2 containing potassium fluoride) samples from 100 healthy participants aged between 18 and 60 years were collected by experienced phlebotomists using 21-gauge vacutainer needles (Becton Dickinson). One set of tubes for all volunteers were sent via PTS while the remaining set of tubes were hand carried to the laboratory. The paired samples transported by PTS and manual courier were compared for 25 biochemistry parameters. The peak acceleration of PTS was also calculated by the data logger and the hemolysis index (HI) of the hemolyzed sample by colorimetry. The threshold point was set at 0.3 g/L of hemoglobin, above which it has the potential to produce interference in the analysis of another test. Paired t-test or Wilcoxon signed-rank test was used to assess the significance of difference between PTS and hand-held samples.
The results were tested for normality of the data using the Shapiro–Wilk test. If values were found normal, then the mean and standard deviation were calculated and analyzed using the Student's t-test. But if the data were not normally distributed, the median with interquartile range was calculated and the Wilcoxon signed-rank test was performed for comparison.
Clinical significance is analyte-specific but by convention, percent average bias of more than ± 10% was considered significant. Few parameters have more stringent clinical acceptable limits (0.075 mmol/L for calcium, 3.0 mmol/L for sodium, 0.3 mmol/L for potassium).[7] Microsoft Office Excel and IBM SPSS statistics were utilized for data entry and their statistical calculation.
In phase II of the study, samples were again collected to verify the efficacy of the corrective action. The sample size, collection procedure, study design, and statistical analysis remained the same.
Ethical Requirement
To carry out this study, permission was granted by the Indira Gandhi Institute of Medical Sciences' (IGIMS) Ethical Committee as per letter no. 1119 dated 04/10/2019. All participants provided their informed consent. We followed the guideline provided by the Helsinki Declaration.
PTS
The PTS system installed at IGIMS is the Telecom Medical tube system, a unit of telecom, Netherlands. The total distance from the blood collection unit to the CBL is 300 m and the average time taken to reach there is 106 seconds. The speed of the carrier throughout the trajectory path remains 3 to 6 m/s. There are four stations at different locations to load and receive the carrier. The length of the PTS carrier is 330 mm and the diameter is 76 mm.[6,10]
Data Logger
A data logger based on MPU 6050 chip was used to measure the acceleration and deceleration in x-, y-, and z-directions. The data logger was put in the pneumatic tube sample transport carrier gently along with blood samples in 5 mL tubes. The carrier was put in the PTS and dispatched from the sample collection area. The data were collected throughout the path. At the end of the run, the data logger was taken out and switched off to stop the recording.
Phlebotomy
Blood samples were collected by experienced phlebotomists according to the recommendation of the Clinical and Laboratory Standards Institute (CLSI). The same collection protocol was followed in both phase I and phase II of the study. From all donors duplicate serum and plasma glucose samples were collected using Becton Dickinson tubes and vacutainers. One set of tubes was bar-coded mechanically by BC.ROBO-888 (Techno Medica Company Limited) and the second set of tubes was labeled manually for manual courier transport to the CBL, IGIMS. The bar-coded samples were sorted by a fully automated sorter machine (ATRAS) and then sent to the specific destination through the telecom PTS system. After the arrival of the carrier at the CBL station, the samples are taken out manually and placed in the ATRAS for automated sorting; so that, different samples could be segregated and sent to their respective instrument, e.g., routine chemistry, immunoassay, and other specialized tests. All the samples, both PTS and manually transported, were then further processed for centrifugation, analysis, and archiving. Analysis was done on biochemistry automated analyzer Beckman Coulter AU 5800 and DxC 700 AU (United States). The quality control and calibration of the instruments were maintained, using Bio-Rad internal QC and Beckman Coulter calibrator materials. Samples of the same donor were run on a single instrument. A total of 25 parameters were compared between samples carried by PTS and human couriers.
Results
Analytes having normal distribution after the Shapiro–Wilk test are compared for their significance by the Student's t-test. Those parameters having nonnormal distribution are compared by the Wilcoxon signed-rank test.
For the phase I of the study, two sets of data for PTS and handheld samples from100 volunteers each (total 400 tubes = 200 serum and 200 plasma for glucose) were exploited for analysis. Samples having very high values were not considered in the calculation. in ►Table 1 statistically calculated results of phase I of the study are mentioned and compared. Results of potassium, LDH, AST, and phosphorus were raised in PTS samples having a significant difference; the p-values were 0.000, 0.001, 0.025, and 0.047, respectively. These are all hemolysis-manifesting parameters. The mean average difference of LDH and potassium were clinically significant also. In addition, 9% of PTS samples and 2.5% of hand-carried samples were hemolyzed in phase I. Total bilirubin and direct bilirubin were significantly raised in hand-carried samples having p-values of 0.032 and 0.47 respectively, but the difference was clinically nonsignificant. This may be due to increased hemolysis leading to decreased azobilirubin color development in van den Bergh reaction. These all results verified that hemolysis was occurring in PTS samples in high frequency as compared with the manually carried samples.
Parameters | Units | n | Mean ± SD/median (IR) | (PTS—manual) | p-Value (paired t-test or Wilcoxon signed rank) | ||
---|---|---|---|---|---|---|---|
PTS transport | Manual transport | Meandifference | SDdifference | ||||
Biochemical parameters with normal distribution | |||||||
Calcium | mmol/L | 200 | 2.51 ± 0.37 | 2.46 ± 0.34 | 0.05 | 0.04 | 0.402 |
Chloride | mmol/L | 200 | 107 ± 2.62 | 105 ± 2.80 | −2 | 0.02 | 0.095 |
Creatinine | μmol/L | 200 | 84.88 ± 20.54 | 85.30 ± 19.78 | −0.42 | 0.76 | 0.730 |
High-density lipoprotein | mmol/L | 200 | 1.53 ± 0.22 | 1.53 ± 0.29 | 0 | −0.07 | 0.899 |
Phosphorus | mmol/L | 200 | 1.09 ± 0.09 | 1.0 ± 0.03 | −0.05 | −0.06 | 0.047a |
Potassium | mmol/L | 200 | 4.69 ± 0.69 | 4.12 ± 0.78 | −0.57 | −0.09 | 0.000a |
Sodium | mmol/L | 200 | 139 ± 1.4 | 138 ± 1.6 | 1.0 | −0.2 | 0.061 |
Urea | mmol/L | 200 | 2.51 ± 0.37 | 2.46 ± 0.34 | 0.05 | 0.04 | 0.402 |
Biochemical parameters with nonnormal distribution | |||||||
Alanine aminotransferase | U/L | 200 | 18 (10) | 19 (10.9) | −1.0 | −0.9 | 0.283 |
Albumin | μmol /L | 200 | 65.22 (10.12) | 65.20 (10.16) | 0.02 | −0.4 | 0.464 |
Alkaline phosphatase | U/L | 200 | 72.3 (18) | 70.5 (18.9) | −1.8 | −0.9 | 0.377 |
Amylase | U/L | 200 | 20.7 (11.6) | 20.2 (11.3) | 0.5 | −0.3 | 0.398 |
Aspartate aminotransferase | U/L | 200 | 32 (19) | 28 (17) | −4 | −3 | 0.025a |
Direct bilirubin | μmol/L | 200 | 1.88 (0.86) | 1.97 (0.97) | 0.07 | −0.11 | 0.043a |
Glucose | mmol/L | 200 | 4.94 (1.02) | 4.93 (1.01) | 0.01 | 0.01 | 0.139 |
Iron | μmol/L | 200 | 10.06 (8.54) | 10.06 (8.58) | 0 | 0.04 | 0.999 |
LDH | U/L | 200 | 347 (345) | 262 (157) | −84 | −18 | 0.001a |
Low-density lipoproteins | mmol/L | 200 | 2.44 (0.66) | 2.40 (0.70) | 0.04 | −0.04 | 0.652 |
Magnesium | mmol/L | 200 | 0.798 (0.06) | 0.801 (0.04) | −0.003 | 0.02 | 0.756 |
Protein | g/L | 200 | 80.44 (7.23) | 80.42 (7.93) | 0.02 | 0.30 | 0.867 |
Total bilirubin | μmol/L | 200 | 6.54 (4.23) | 6.90 (4.45) | −0.36 | −0.31 | 0.032a |
Total cholesterol | mmol/L | 200 | 4.37 (1.24) | 4.39 (1.34) | −0.02 | −0.10 | 0.741 |
Triglyceride | mmol/L | 200 | 1.09 (0.82) | 1.11 (0.83) | 0.04 | −0.01 | 0.685 |
Uric acid | μmol/L | 200 | 260 (120.3) | 258 (127.0) | 2.0 | −6.7 | 0.398 |
Hemolysis index (> 0.3 gm/L of hemoglobin) | |||||||
Hemolysis | No. (%) | 200 | 18 (9) | 5 (2.5) |
Abbreviations: PTS; pneumatic transport system; SD, standard deviation.
Note: Analytes manifesting hemolysis potassium, LDH, and AST showing significant difference; total bilirubin and direct bilirubin also show significant difference between the samples.
a p-Value < 0.05, hence significant.
Results of phase II of the study are tabulated in ►Table 2, where values of a total of 200 paired samples (PTS and hand transported) are compared. The difference of all the paired samples are statistically insignificant (p > 0.05) except for the sodium, potassium, and AST having p equal to 0.045, 0.012, and 0.042, respectively. However, the differences were statistically significant but within clinically acceptable limits. In addition, 2.5% PTS samples and 2% of hand-carried samples were hemolyzed in phase II. The differences of all other parameters that manifest hemolysis were nonsignificant. This shows that among PTS samples, the hemolysis frequency is settled to a normal level.
Parameters | Units | n | Mean ± SD/median (IR) | (PTS—manual) | p-Value (paired t- test or Wilcoxon signed rank) | ||
---|---|---|---|---|---|---|---|
PTS transport | Manual transport | Mean difference | SD difference | ||||
Biochemical parameters with normal distribution | |||||||
Calcium | mmol/L | 200 | 2.47 ± 0.39 | 2.41 ± 0.35 | 0.06 | 0.04 | 0.421 |
Chloride | mmol/L | 200 | 104 ± 2.82 | 105 ± 2.80 | −1 | 0.02 | 0.085 |
Creatinine | μmol/L | 200 | 85.45 ± 25.76 | 84.98 ± 26.54 | 0.47 | −0.78 | 0.930 |
High-density lipoprotein | mmol/L | 200 | 1.47 ± 0.24 | 1.47 ± 0.29 | 0 | −0.05 | 0.999 |
Phosphorus | mmol/L | 200 | 1.07 ± 0.05 | 1.08 ± 0.06 | −0.01 | −0.01 | 0.376 |
Potassium | mmol/L | 200 | 4.14 ± 0.50 | 4.34 ± 0.64 | −0.2 | −0.14 | 0.042a |
Sodium | mmol/L | 200 | 138 ± 1.4 | 139 ± 1.6 | −1.0 | −0.2 | 0.045a |
Urea | mmol/L | 200 | 3.88 ± 0.15 | 3.85 ± 0.18 | 0.03 | −0.03 | 0.377 |
Biochemical parameters with nonnormal distribution | |||||||
Alanine aminotransferase | U/L | 200 | 23 (11) | 23 (11.9) | 0.0 | −0.9 | 0.983 |
Albumin | μmol /L | 200 | 69.21 (10.38) | 69.20 (10.38) | 0.01 | 0 | 0.764 |
Alkaline phosphatase | U/L | 200 | 95 (24) | 94.5 (24.9) | −0.5 | −0.9 | 0.677 |
Amylase | U/L | 200 | 32.7 (20.3) | 32.4 (20.6) | 0.3 | −0.3 | 0.798 |
Aspartate aminotransferase | U/L | 200 | 28 (16) | 29.6 (16.5) | −1.6 | −0.5 | 0.042a |
Direct bilirubin | μmol/L | 200 | 2.05 (0.86) | 1.96 (0.97) | 0.09 | −0.11 | 0.043a |
Glucose | mmol/L | 200 | 4.94 (1.02) | 4.93 (1.01) | 0.01 | 0.01 | 0.139 |
Iron | μmol/L | 200 | 10.06 (8.54) | 10.06 (8.58) | 0 | 0.04 | 0.999 |
LDH | U/L | 200 | 214 (52) | 198 (60) | 16 | −8 | 0.218 |
Low-density lipoproteins | mmol/L | 200 | 2.74 (0.67) | 2.72 (0.70) | 0.02 | −0.03 | 0.765 |
Magnesium | mmol/L | 200 | 0.803 (0.06) | 0.801 (0.04) | 0.002 | 0.02 | 0.768 |
Protein | g/L | 200 | 81.44 (9.23) | 81.42 (8.98) | 0.02 | 0.25 | 0.967 |
Total bilirubin | μmol/L | 200 | 6.82 (3.23) | 6.74 (2.45) | 0.08 | 0.78 | 0.06 |
Total cholesterol | mmol/L | 200 | 4.64 (1.17) | 4.63 (1.16) | 0.01 | 0.01 | 0.843 |
Triglyceride | mmol/L | 200 | 1.40 (0.82) | 1.40 (0.83) | 0 | −0.01 | 0.985 |
Uric acid | μmol/L | 200 | 261 (180.3) | 260 (197.0) | 1 | −16.7 | 0.286 |
Hemolysis index (> 0.3 gm/L of hemoglobin) | |||||||
Hemolysis | No. (%) | 200 | 5 (5) | 4 (4) |
Abbreviations: PTS; pneumatic transport system; SD, standard deviation.
a p-Value < 0.05, hence significant.
The results of the data logger are shown in ►Figs. 1,2, and 3 and ►Table 3. The g-forces experienced by the samples through PTS and handling during manual transport were measured. The absolute vector sums (x-, y-, and z-axes) of acceleration values recorded during the transit were plotted against the data points taken at an interval of 1 second. In the PTS experiment, the g-force is collected both in phase I (high pressure/high-speed setting) and phase II (reduced pressure/low-speed setting). The peak acceleration in PTS samples of phase I was 15.7 g, phase II 11.2 g, and in handheld (both phases) samples 1.2 g.

- The measured g-forces during the run through pneumatic tube at phase I.
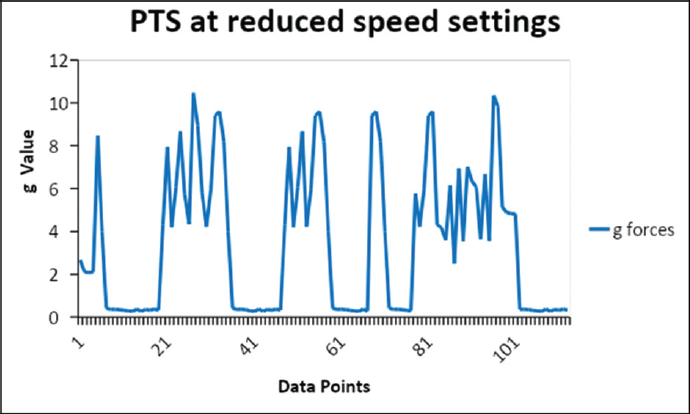
- The measured g-forces during the run through pneumatic tube in phase II.
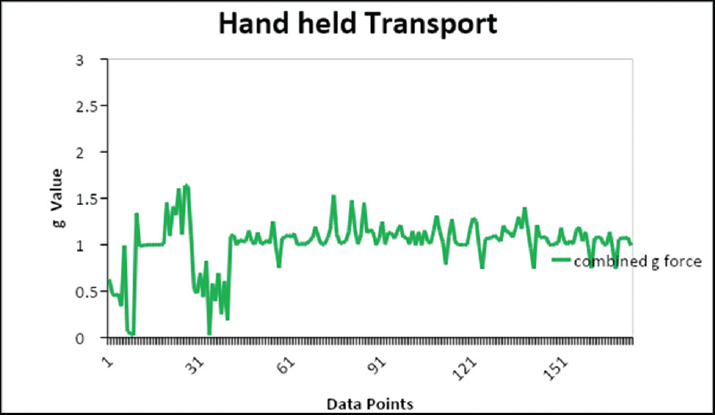
- The measured g-forces during the manual hand-held transport.
Run | Maximum g-forces (g) |
---|---|
Phase I: PTS at high-speed setting | 15.7 |
Phase II: PTS at reduced speed settings | 11.2 |
Hand held | 1.6 |
Abbreviation: PTS; pneumatic transport system.
►Table 4 shows the time taken by the carrier to reach the CBL. For PTS samples in phase I, it was 61 seconds, in phase II, 106 seconds, and for handheld (both phases) samples, 177 seconds.
Run | Traverse time (s) |
---|---|
Phase I: PTS at high-speed setting | 61 |
Phase II: PTS at reduced speed settings | 106 |
Hand held | 177 |
Abbreviation: PTS; pneumatic transport system; s, seconds.
Discussion
In this era of laboratory automation, it is always advantageous to embrace the latest technology to improve efficiency. But any new methodology should be validated so that it is not jeopardizing the quality of test results. Hence, we assessed our telecom PTS to evaluate its potential effects on routine biochemical parameters. Although we were able to test all analytes being performed in CBL including immunoassay parameters, but previous studies reported only inconsistent findings regarding hemolysis. Though hemolysis may affect the entire laboratory methods, photometry is predominantly affected. So, only routine biochemistry parameters were selected for this study.
The parameters manifesting hemolysis are LDH, HI, AST, and K+ (potassium). In phase I, the values of potassium, LDH, AST, and phosphorus were higher in PTS samples as compared with manually carried samples; the difference was significant having p-values 0.000, 0.001, 0.025, and 0.047, respectively. The differences were within the clinically acceptable limit for AST and phosphorus, but for potassium and LDH, they were larger than clinically acceptable limits. The HI was also raised in PTS samples as 9% of them were hemolyzed as compared with 2.5% of manual samples. This corroborated with the complaints of reporting of potassium and LDH by clinicians after installation of PTS in the biochemistry department. Transportation time was raised from 61 to 106 seconds in phase II but still less than hand transport time, which was 177 seconds.
In phase II of the study, there was no statistically significant difference between hand- and PTS-transported samples for almost all the biochemical parameters except for sodium, potassium, and AST. Furthermore, none of them manifested clinically significant difference between the paired samples. All the differences were within the clinically acceptable limits. This confirmed significant reduction of hemolysis after pressure adjustment.
The peak acceleration observed by PTS samples was 15.7 g before the pressure change and 11.2 g after the correction. The hand-held samples showed a maximum acceleration of 1.6 g. Gils et al in their study found that in hand held it was 5 g and in the PTS system it was 13 g.[5] The speed in our telecom PTS was found to be varied from 3 to 6 m/s, whereas Streichert et al mentioned the highest speed of 2.5 m/s, the speed of the carrier reported by Lee et al was 10 m/s, which was higher than what we observed (3–6 m/s) in our study.[1]
Streichert et al in their study determined the hemolysis thresholds by the utilizing results of data loggers in pneumatic tube systems. They found comparable results for PTS samples, which were 15 g, but for hand-transported samples the peak acceleration was 9 g. This variation in hand-transported samples may be due to the mode of manual transportation like the transportation box or the transportation wagon. Streichert et al additionally found clinically significant changes between samples for K+ and LDH (changes beyond the acceptable deviation of quality control—4.5% for K+ and 9% for LDH) and these changes diminished with the reduction in speed and decreasing area under the curve (AUC).[19] Gils et al's findings corroborated with this for Department A but could not find a positive correlation between PTS speed and AUC. They inferred that increased cell lysis could be due to increasing vector sums of acceleration in hematological patients having unstable blood components.[5]
Amann et al demonstrated the effects of increasing acceleration on cellular integrity. Results showed a positive correlation between hemolysis and increasing acceleration of PTS lines.[9,20] Koçak et al found no significant effects imparted by the PTS on blood cell counts, erythrocyte sedimentation rate (ESR), prothrombin time, or activated partial thromboplastin time results. Hence, they concluded that PTS in their hospital can be used reliably for transporting blood samples without disturbing blood cell counts, ESR, or several types of coagulation tests.[16]
Pupek et al validated their PTS for biochemistry, hematology, and blood gas samples collected in ABG syringes and capillary tubes, all the samples were collected in pairs.[11] The difference of two samples for some parameters (alkaline phosphatase, total bilirubin, hemoglobin) was statistically significant but the total bias was less than 2%. Similarly, no difference for blood gas parameters was observed (except deoxyhemoglobin, potassium, total hemoglobin); the bias was less than 4%. After this result, they removed the GEM 4000 analyzer from the neonatal intensive care unit and started reporting blood gas from capillary tube samples transported to the core laboratory via PTS. The TAT for blood gas analysis was acceptable for the neonatologist. So, the installation of PTS not just curtailed the need for point-of-care instruments but also helped in maintaining the TAT.[11,12,21]
A study by Lee et al concluded that no statistically significant differences existed between PTS- and hand-transported samples for complete blood count, white blood cell differential count, and ESR.[1] Results of their study correspond well with those of many similar works which reported that PTS does not affect hematology parameters.[12,22,23] In Lee et al's work, there was a statistically significant difference for mean platelet volume (p = 0.001). The mean difference was 0.3 fL, which was lower in samples transported via PTS than in samples transported via a hand-delivered method. But this difference was likely to be clinically acceptable. Among biochemical parameters, the differences in AST, LDH, and HI were statistically significant but there was no difference in potassium values between hand- and PTS-transported samples. Several other researchers reported raised LDH and K+ in PTS samples due to acceleration and deceleration.[22,24,25]
Tiwari et al worked on paired samples from three phases—(1) long-distance and high speed (225 m at 3 m/s), (2) short distance and high speed (115 m at 3 m/s), and (3) short distance and slow speed (115 at 2 m/s). Phases (1) and (2) showed a raised level of all three indices of hemolysis—hemoglobin, K +, and LDH—in the PTS samples. However, the third or short distance and slow speed phase demonstrated no hemolysis in the PTS arm.[9]
The effect of transportation by PTS on platelets has previously been investigated. Using impedance platelet aggregometry TRAP and aspirin tests, significant influences were demonstrated in 50 patients scheduled for coronary artery bypass surgery under oral therapy with 100 mg/day acetylsalicylic acid by PTS.[17]
Higgins in his article “Pneumatic tube transport of blood samples—an update” summarized that pneumatic-induced hemolysis is system-dependent and each system should be assessed and monitored frequently. He preferred miniaturized data loggers over paired sample tests as it is a simple and convenient method for individual PTS assessment. No effect due to PTS on pH and pCO2 analysis but erroneous pO2 values were cited by the author. Serum samples are more prone to hemolysis than plasma samples. He cautioned the transport of patient samples with high-white-cell-count malignancies.[26]
The limitation of our study is that individual confounding factors accountable for hemolysis, which included pressure applied, acceleration of samples, cushioning like sponge rubber inserts, and an ideal technique of phlebotomists, were not compared separately. The threshold level of pressure for hemolysis was not calculated. Although our PTS has three more stations in Hematology, Microbiology, and Pathology, but our study is restricted only to the Biochemistry department. Hematological or microbiological parameters were not included in the study. Those parameters which required strict stringent conditions like immediate separation and cooling after the collection were also not included in the present study. These samples should have a separate processing plan, but we are handling all samples by the same route. A study should also be performed to find any difference in results between samples having different RBC membrane defects and other clinical states that aggravate hemolysis.
There is no proposed recommendation for quality assessment of PTS that could be followed worldwide. So every user should work on developing quality-control methods for their PTS. Nybo et al reviewed the studies on PTS and concluded that evaluation of the PTS is essential at the time of installation to nullify the potential preanalytical component from the results.[27]
Conclusion
In the present study increased hemolysis was found in PTS samples as evidenced by the significant statistical difference in the values of HI, LDH, and potassium between PTS and manually carried samples. This difference is larger than the clinically acceptable limits of the respective parameter. Alterations were done in the configuration of PTS like lowering the blower pressure, programming of a shorter path, and use of cushioning in carriers and below the station. Consequently, there was no statistical difference in the values of hand-carried and PTS samples for biochemistry parameters except a few which are clinically acceptable. PTS showed no observable impact on the biochemistry results of blood samples after the adjustment was done. The complaints of hemolysis have drastically reduced.
Hence, PTS validation is not just mandatory for every institute but its specific configurations should be monitored at frequent intervals. All users should be acquainted with its potential effects on blood sample integrity and remain vigilant to take measures consequently.
Conflict of Interest
None declared.
References
- Effects of one directional pneumatic tube system on routine hematology and chemistry parameters; a validation study at a tertiary care hospital. Pract Lab Med. 2017;9:12-17.
- [CrossRef] [PubMed] [Google Scholar]
- European Federation for Clinical Chemistry and Laboratory Medicine Working Group for Preanalytical Phase. Preanalytical quality improvement. In pursuit of harmony, on behalf of European Federation for Clinical Chemistry and Laboratory Medicine (EFLM) Working group for Preanalytical Phase (WG-PRE) Clin Chem Lab Med. 2015;53(03):357-370.
- [CrossRef] [Google Scholar]
- The detection and prevention of errors in laboratory medicine. Ann Clin Biochem. 2010;47(Pt 2):101-110.
- [CrossRef] [PubMed] [Google Scholar]
- Preanalytical quality improvement: from dream to reality. Clin Chem Lab Med. 2011;49(07):1113-1126.
- [CrossRef] [Google Scholar]
- Use of clinical data and acceleration profiles to validate pneumatic transportation systems. Clin Chem Lab Med. 2020;58(04):560-568.
- [CrossRef] [PubMed] [Google Scholar]
- Working Group for Preanalytical Phase (WG-PRE) and European Federation of Clinical Chemistry and Laboratory Medicine (EFLM). The role of European Federation of Clinical Chemistry and Laboratory Medicine Working Group for Preanalytical Phase in standardization and harmonization of the preanalytical phase in Europe. Ann Clin Biochem. 2016;53(Pt 5):539-547.
- [CrossRef] [PubMed] [Google Scholar]
- The impact of a pneumatic tube and computerized physician order management on laboratory turnaround time. Ann Emerg Med. 2008;51(02):181-185.
- [CrossRef] [PubMed] [Google Scholar]
- Assessing safety of pneumatic tube system (PTS) for patients with very low hematologic parameters. Med Sci Monit. 2016;22:1329-1333.
- [CrossRef] [PubMed] [Google Scholar]
- Speed of sample transportation by a pneumatic tube system can influence the degree of hemolysis. Clin Chem Lab Med. 2011;50(03):471-474.
- [CrossRef] [PubMed] [Google Scholar]
- 2009 Accessed September 24, 2021 at. https://www.telecomtubesystems.com/Telecom/download/3htm2009-design-good-practice-guide.pdf
- Comparison of pneumatic tube system with manual transport for routine chemistry, hematology, coagulation and blood gas tests. Clin Chem Lab Med. 2017;55(10):1537-1544.
- [CrossRef] [PubMed] [Google Scholar]
- Pneumatic tube delivery system for blood samples reduces turnaround times without affecting sample quality. J Emerg Nurs. 2006;32(02):139-143.
- [CrossRef] [PubMed] [Google Scholar]
- Automated approaches to rapid-response testing. A comparative evaluation of point-of-care and centralized laboratory testing. Am J Clin Pathol. 1995;104(04, Suppl 1):S18-S25.
- [Google Scholar]
- The effect of pneumatic tube systems on the hemolysis of biochemistry blood samples. J Emerg Nurs. 2017;43(03):255-258.
- [CrossRef] [PubMed] [Google Scholar]
- The preanalytical influence of two different mechanical transport systems on laboratory analysis. Clin Chem Lab Med. 2011;49(08):1379-1382.
- [CrossRef] [PubMed] [Google Scholar]
- The effects of transport by pneumatic tube system on blood cell count, erythrocyte sedimentation and coagulation tests. Biochem Med (Zagreb). 2013;23(02):206-210.
- [Google Scholar]
- Pre-analytical effects of pneumatic tube transport on impedance platelet aggregometry. Platelets. 2009;20(07):458-465.
- [CrossRef] [PubMed] [Google Scholar]
- Pneumatic tube system induced haemolysis: assessing sample type susceptibility to haemolysis. Ann Clin Biochem. 2004;41(Pt 3):237-240.
- [CrossRef] [PubMed] [Google Scholar]
- Determination of hemolysis thresholds by the use of data loggers in pneumatic tube systems. Clin Chem. 2011;57(10):1390-1397.
- [CrossRef] [PubMed] [Google Scholar]
- Effect of acceleration forces during transport through a pneumatic tube system on ROTEM® analysis. Clin Chem Lab Med. 2012;50(08):1335-1342.
- [CrossRef] [PubMed] [Google Scholar]
- Extending laboratory automation to the wards: effect of an innovative pneumatic tube system on diagnostic samples and transport time. Clin Chem Lab Med. 2017;55(02):225-230.
- [CrossRef] [PubMed] [Google Scholar]
- Effects of a pneumatic tube system on routine and novel hematology and coagulation parameters in healthy volunteers. Arch Pathol Lab Med. 2007;131(02):293-296.
- [CrossRef] [PubMed] [Google Scholar]
- Management of preanalytical phase for routine hematological testing: is the pneumatic tube system a source of laboratory variability or an important facility tool? Int J Lab Hematol. 2014;36(04):e37-e40.
- [CrossRef] [PubMed] [Google Scholar]
- Preanalytical phase–a continuous challenge for laboratory professionals. Biochem Med (Zagreb). 2012;22(02):145-149.
- [CrossRef] [PubMed] [Google Scholar]
- Evaluation of pneumatic-tube system for delivery of blood specimens. Clin Chem. 1971;17(12):1160-1164.
- [CrossRef] [PubMed] [Google Scholar]
- Pneumatic tube transport of blood samples – an update. October 2015 Accessed September 24, 2021 at: https://acutecaretesting.org/en/articles/pneumatic-tube-transport-of-blood-samples-an-update
- [Google Scholar]
- Sample transportation - an overview. Diagnosis (Berl). 2019;6(01):39-43.
- [CrossRef] [PubMed] [Google Scholar]