Translate this page into:
Resveratrol attenuates malathion-induced liver damage by reducing oxidative stress
Address for correspondence: Prof. Mohammad Reza Salahshoor, Department of Anatomical Sciences, Medical School, Kermanshah University of Medical Sciences, Daneshgah Ave., Taghbostan Kermanshah, Iran. E-mail: reza.salahshoor@yahoo.com
-
Received: ,
Accepted: ,
This is an open access journal, and articles are distributed under the terms of the Creative Commons Attribution-NonCommercial-ShareAlike 4.0 License, which allows others to remix, tweak, and build upon the work non-commercially, as long as appropriate credit is given and the new creations are licensed under the identical terms.
This article was originally published by Wolters Kluwer - Medknow and was migrated to Scientific Scholar after the change of Publisher.
Abstract
BACKGROUND:
Malathion is an organophosphate insecticide which disrupts the antioxidant system of the body. Resveratrol is a phytoestrogen and antioxidant of the red grape.
AIM AND OBJECTIVE:
This study was designed to evaluate the effects of resveratrol against toxic effects of malathion to the liver of rats.
MATERIALS AND METHODS:
In this study, 48 male rats were randomly assigned to 8 groups: control normal (saline) and malathion control-treated groups (50 mg/kg), resveratrol groups (2, 8, and 20 mg/kg), and malathion + resveratrol-treated groups (2, 8, and 20 mg/kg). Treatments were administered intraperitoneally daily for 14 days. Griess technique was assessed for determined serum nitrite oxide level. Aspartate aminotransferase, alanine aminotransferase, and alkaline phosphatase concentrations were determined for liver functional disturbances. In addition, thiobarbituric acid reactive species, antioxidant capacity, the diameter of hepatocytes, and the central hepatic vein (CHV) were investigated.
RESULTS:
Malathion administration significantly improved liver malondialdehyde (MDA) and nitrite oxide level, the mean diameter of CHV and hepatocyte, and liver enzymes and decreased tissue ferric-reducing ability of plasma (FRAP) level compared to the normal control group (P < 0.01). The resveratrol and resveratrol + malathion treatments at all doses significantly reduced the mean diameter of hepatocyte and CHV, liver enzymes, kidney MDA, and nitrite oxide levels and increased tissue FRAP level compared to the malathion control group (P < 0.01).
CONCLUSION:
It seems that resveratrol administration improved liver injury induced by malathion in rats.
Keywords
Herbal medicine
liver injury
malathion
oxidative stress
Introduction
Organophosphates are a bunch of pesticides that are widely used in agriculture, veterinary medicine, industry, and homes. These compounds are one of the most common causes of poisoning and their complications are either acute or chronic.[1] Organophosphate poisoning is one of the global health problems and has been reported as the third and leading cause of death from poisoning in Iran.[2] Organophosphates enhance acetylcholine by inhibiting cholinesterase and hence stimulate nicotine and muscarinic receptors.[3] Malathion, a kind of organophosphate, is widely used as an insecticide and pesticide and also as a vermicide in the veterinary medicine.[4] It seems that the toxic effects of some organophosphates are not limited to cholinesterase inhibitors; however, some changes are observed due to the increase in acetylcholine, such as the damage to cell membranes, the production of free radicals, and the disturbances in the body's antioxidant system.[5] There is a balance between the production and removal of free radicals in natural conditions, and oxidative stress is caused due to an imbalance in this process.[6] Malation (C10H19O6PS2) is one of the most widely used insecticides. It is a thick, oily, and dark-brownish yellow liquid that is extensively used as a pesticide in agriculture.[7] It is a phosphoric acid derivative and is one of the oldest groups of phosphoric insecticides.[8] According to the results of the study by Fortunato et al., it was found that the production of free radicals and oxidative stress in the brain is induced by malathion and it also increases antioxidant enzyme activity.[9] Organophosphates can cause changes in cellular antioxidant systems and membrane lipid peroxidation by producing free radicals.[10] The liver is considered as an important source of toxic detoxification due to its abundant antioxidants.[11] It has been proven that reactive oxygen species (ROS) in organophosphate toxicity, specifically malathion, is involved in humans.[12] Herbal biological substances that form a branch of modern pharmacotherapy show fewer side effects in patients; hence, the attempts to discover any natural product are of clinical importance in this field.[13] Resveratrol (3,5,4′-trihydroxy-trans-stilbene), a type of phytoalexin, is found in at least 72 plant species.[14] One of the resveratrol resources is the skin of grapes, which contains 100–50 μg/g of wet weight and these results in a relatively high resveratrol concentration in wines.[15] Resveratrol is a phytotoxic polyphenol derivative from plants that are produced by the stilbene synthase in reaction to environmental stresses, such as climate change, ozone exposure, sunlight, heavy metals, and infectious agents such as pathogen microorganisms.[16] It has been proven that resveratrol has anti-inflammatory,[17] antioxidant,[18] and antidiabetic effects.[19] Resveratrol can reduce lipid peroxidation and DNA damage by purifying hydroxyl radicals.[20] This substance is metabolized in the liver by the liver Phase II enzymes to the trans-resveratrol-3-O-glucuronide in water and trans-resveratrol-3-sulfate after the absorption of resveratrol in humans and is often excreted through the urine.[21] It seems that resveratrol also has anti-inflammatory activity and directly inhibits cyclooxygenase-2 (COX-2) activity and applies its anti-inflammatory activity in a variety of ways which are mostly focused on COX-2 and COX inhibiting.[22] Not only does a resveratrol inhibit the COX-2 and COX-1 expression, but also it can reduce the production of prostaglandin E2 and the form ROS from lipopolysaccharide-induced microglia activation by suppressing the activity of nuclear factor-kappa β (NF-Kβ) and I-Kβ kinase.[23] The purpose of this study is to investigate the effects of resveratrol on malathion-induced liver injury in male rats due to the toxic effects of malathion and the beneficial and multiple properties of resveratrol, particularly its antioxidant properties, and since the effects of resveratrol on malathion-induced liver injury has not been clarified yet.
Materials and Methods
Animals
Animal studies were conducted according to the guidelines for the care and handling of animals prepared by the Iranian Ministry of Health. Sixty-four male Wistar rats (weighing 220–250 g) were purchased from Pastor Institute of Iran. The animals were housed in standard cages (three per cage) and control conditions at 23°C ± 2°C and exposed to 12-h light/dark cycle, in Kermanshah University of Medical Sciences animal care facilities for a period of 1 week before testing and exposing to environmental and climatic conditions. The animals had free access to water and food during this period. All investigations conformed to the ethical and humane principles of the research and were approved by the Ethics Committee of Medical Sciences (ethics certificate No. 1396.612).[6]
Study groups and treatment
Forty-eight male rats were randomly divided into 8 groups and 6 rats were placed in each group: first group – the normal control group that received normal saline (through interperitoneal injection) equivalent to the amount of experimental groups; l second group – the control group of malathion (in this group, each animal received 50 mg/kg single-dose course of malathion, intraperitoneally once a day for 14 consecutive days) (the solvent of malathion was normal saline);[24] third to fifth groups – the resveratrol administration groups (in these groups, each animal respectively received 2, 8, and 20 mg/kg of resveratrol intraperitoneally, for 14 days at 10 am;[14] and sixth to eighth groups – malathion + resveratrol administration groups (in these groups, each animal received a single dose of 50 mg/kg malathion in order to induce liver damage, and then, they respectively received 2, 8, and 20 mg/kg) of resveratrol intraperitoneally for 14 days, at 10.5 am.[1424]
Dissection and sampling
Twenty-four hour after the last injection, rats related to each group were placed one after another in a plastic container in a packet of cotton covered with ether, and after a short time due to inhalation of ether fume, they were anesthetized. Venipuncture from the animals' hearts (right ventricle) was done using a 5cc syringe. The blood sample was incubated for 15 min at 37°C to clot. Then, the clotted blood was then centrifuged for 15 min at 3000 rpm until the serum was separated. The separated serum was stored at the temperature of −70°C until some biochemical parameters of the liver, nitric oxide (NO) level, oxidative stress, and antioxidant capacity level were measured. Then, animals' liver was removed and was maintained in 10% formalin solution for histological and morphometric investigations.[14]
Oxidative stress
In order to assess oxidative stress, the thiobarbituric acid reactive species were measured using malondialdehyde (MDA) as the last product of lipid peroxidation in liver tissue by colorimetric analyze. In brief, 1400 μl of acetic acid (Sigma, USA), 1400 μl of TBA (Sigma, USA), and 1400 μl of sodium dodecyl sulfate (Sigma, USA) were added to 100 μl of liver homogenate, and the mixture was animated for 50 min. Four milliliters of 1-butanol (Sigma, USA) was added to the combination and vortexed 2 min through centrifugation at 5000 rpm for 15 min. The absorbance of the higher layer was measured at 532 nm (Spectro, Germany), and sequential concentrations of tetraethoxypropane (Sigma, USA) were used as the external standard. The antioxidant capacity of the liver was measured using ferric-reducing ability of plasma (FRAP) assay. The FRAP substance consisted of 30 ml of acetate buffer (Sigma, USA) and 1.5 ml chloride ferric (Sigma, USA). In brief, 60 μl of kidney homogenate was added to 1.5 ml of newly prepared FRAP substance (Sigma, USA) in a test tube and incubated at 37°C for 10 min. The absorbance of the blue-colored complex was read against a blank at 593 nm. Sequential concentrations of FeSO4.7H2O (Sigma, USA) were used as an external standard.[25]
Enzymes
The liver was split and turned into a uniform solution. To separate the biological enzymes, the obtained solution was centrifuged at 10,000 rpm for 15 min twice. The supernatant was separated to measure the enzymes. Alanine aminotransferase (ALT) and aspartate aminotransferase (AST) actions were examined by the method of Reitman and Frankel. Alkaline phosphatase (ALP) actions were determined according to the procedure set out in the practical laboratory manual.[11]
Serum nitric oxide assay
To measure concentration of nitrite in the serum, after defreezing the serum samples, in this examine, supernatant (400 μl) was deproteinized by zinc sulfate (6 mg zinc sulfate powder was mixed with 400 μL serum and vortexed for 1 min) with centrifugation. 100 μl supernatant was taken, and 100 μl vanadium chloride, 50 μl N-(1-naphthyl) ethylenediamine dihydrochloride, and 50 μl sulfonamide solutions were added. Sodium nitrite (0.1 M) was used for the standard curve, and increasing concentrations of sodium nitrite (5, 10, 25, 50, 75, and 100 μM) were prepared. The Griess solution was added to all microplate containing sodium nitrite and supernatant and was read through an ELISA reader (stat fa × 100, USA) at the wavelength of 540 nm.[20]
Morphologic studies and histopathological examinations
In order to the histological evaluation of the hepatic buildings, the inferior 1-cm-long part of the right lobe of the liver in transverse pieces was removed, washed in saline, and fixed in 10% formalin, dehydrated in ascending concentration ethanol, cleared in xylene, and then embedded by paraffin. Thin sections (4 mm) were cut using a microtome (Leica RM 2125, Germany) and marked with hematoxylin and eosin. For each hepatocyte, the full cellular area was measured. The hepatocyte outline was measured after capturing an image with a ×40 objective. The maximum and minimum axis was measured in the drawing of each hepatocyte for measuring the mean axis. At least 50 hepatocytes from each zone were measured in each liver. A separate measurement for the central hepatic vein (CHV) was performed using the same assay. The planning was examined with an Olympus BX-51T-32E01 research microscope connected to a DP12 Camera with a 3.34-million pixel resolution and Olysia Bio software (Olympus Optical Co. Ltd., Tokyo, Japan).[13]
Statistical analysis
After extracting the information, Kolmogorov–Smirnov test was first conducted to confirm the data compliance of the normal distribution. One-way analysis of variance (one-way ANOVA) was used for statistical analysis, and Tukey's post hoc test was used to determine the difference between the groups. SPSS 16 was used for data analysis, and the results were expressed as mean ± standard error; P < 0.05 was considered statistically significant.
Results
Oxidative stress
The results of the oxidative stress testing in the groups showed that the liver MDA level significantly increased in the malathion control group compared to the normal control group (P < 0.01). The liver MDA level decreased significantly in all malathion + resveratrol groups compared to the malathion control group (P < 0.01). Similarly, malathion significantly decreased the liver tissue FRAP level of the malathion control group in comparison with that of the normal control group (P < 0.01). Administration of resveratrol significantly increased the FRAP level in the liver tissue in all malathion + resveratrol groups compared to the malathion control group (P < 0.01). Tissue ferric-reducing ability of plasma (FRAP) and liver malondialdehyde (MDA) levels in all resveratol groups was not significant compared to the normal control group (P > 0.05) [Figure 1].

- Comparison of malathion, saline, and resveratrol groups of: (a) Liver malondialdehyde level; (b) tissue ferric-reducing ability of plasma level. *Significant increase compared to control normal group (P < 0.01). **Significant decrease compared to malathion control group (P < 0.01). ***Significant decrease compared to malathion control group (P < 0.01). #Significant compared to control normal group (P < 0.01). †Significant increase compared to malathion control group (P < 0.01). ‡Significant increase compared to malathion control group (P < 0.01)
Liver enzymes
Malathion led to a significantly increased in ALT, AST, and ALP enzymes in comparison with the normal control group (P < 0.01). The mean ALT, AST, and ALP enzyme concentration was not significant in all resveratrol groups compared to the normal control group (P > 0.05). Furthermore, in all resveratrol and malathion + resveratrol groups the levels of ALT, AST and ALP enzymes decreased significantly compared to the malathion control group (P < 0.01) [Figure 2].
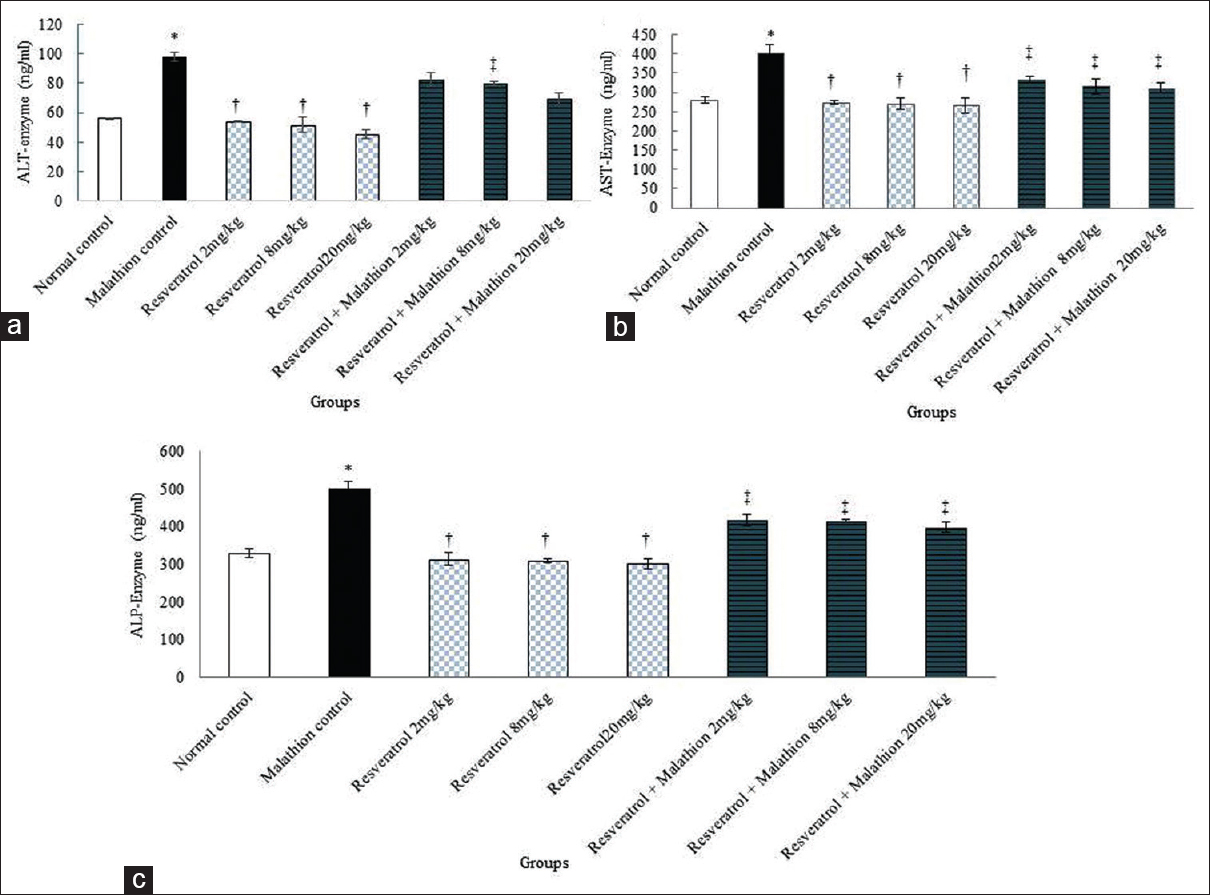
- Effect of malathion, resveratrol, and resveratrol + malathion administration on liver enzymes of 48 rats was equally divided into 8 groups. (a) Alanine aminotransferase enzyme, (b) aspartate aminotransferase enzyme, and (c) alkaline phosphatase enzyme. *Significant increase of enzymes in malathion control group compared to normal control group (P < 0.01). †Significant decrease in all doses of resveratrol groups compared to malathion control group administration (P < 0.01). ‡Significant decrease in all doses of resveratrol + malathion groups compared to malathion control groups (P < 0.01)
Nitrite oxide
The results of blood serum NO measurement showed a significant increase in the malathion control group compared to the control normal group (P < 0.01). The mean nitrite oxide in the blood serum was not significant in all the resveratrol groups compared to the normal control group (P > 0.05). Furthermore, the mean of nitrite oxide in blood serum significantly reduced in resveratrol and malathion + resveratrol at all doses compared to the malathion control group (P < 0.01) [Figure 3].
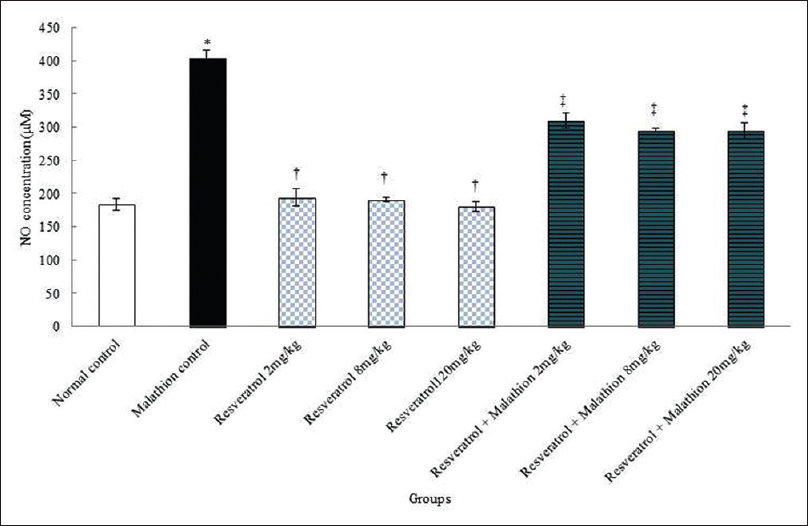
- Effects of resveratrol, malathion, and resveratrol + malathion on the mean nitrite oxide levels. *Significant increase of nitrite oxide in malathion control group compared to normal control group (P < 0.01). †Significant decrease in all doses of resveratrol groups compared to malathion control group (P < 0.01). ‡Significant decrease in all doses of resveratrol + malathion groups compared to malathion control group (P < 0.01)
Morphometric measurements
The mean diameter of hepatocytes and CHV in experimental groups showed a significant difference between the normal control group and the malathion control group (P < 0.01). The mean diameter of hepatocytes and CHV was not significant in all resveratrol groups compared to the normal control group (P > 0.05). Further, resveratrol and malathion + resveratrol significantly reduced the mean diameter of hepatocytes and CHV in all treated groups in comparison with the malathion control group (P < 0.01) [Figure 4].
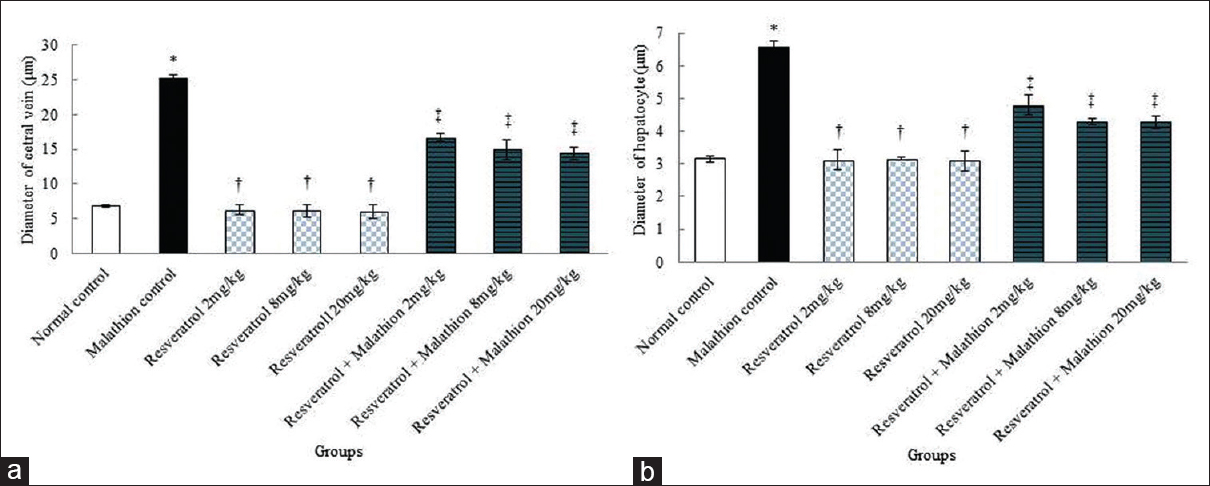
- Effect of malathion, resveratrol, and resveratrol + malathion administration on the central hepatic vein (a) and diameter of hepatocyte (b) diameters. *Significant increase of the mean hepatocytes and central hepatic vein diameters in malathion control group compared to normal control group (P < 0.01). †Significant decrease in all doses of resveratrol groups to malathion control groups (P < 0.01). ‡Significant decrease in all doses of resveratrol + malathion administration compared to malathion control group (P < 0.01)
Histopathological changes
Histological analysis showed normal liver structure in the normal control and resveratrol treatment groups. After treatment with malathion in the malathion control group, the liver showed evident changes and injury. These anomalies included increased white blood cells (inflammation), increased irregularities, sinusoidal dilatation, and the vacuolization of hepatocyte (necrosis). Treatment with malathion + resveratrol at all doses reduced the liver damage caused by malathion toxicity [Figure 5].
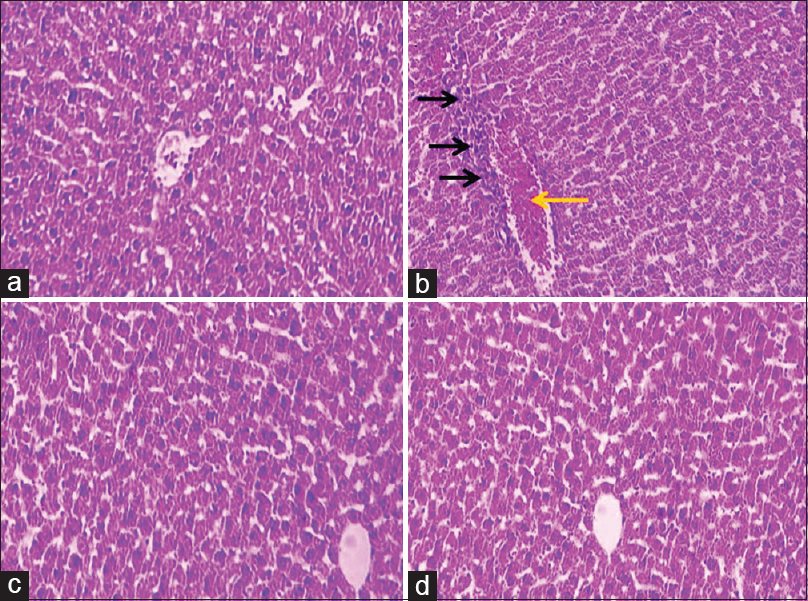
- Microscopic images of liver tissue in mature rats in different groups (five-micron thick sections, H and E, ×100). Micrograph of the liver section in the control normal groups (a), normal liver structure. Micrograph of the liver section in malathion control group (b), increased white blood and macrophage cells (inflammation) (black arrows), and central hepatic vein dilatation and hyperemia (red arrow), due to the oxidative stress caused by malathion. Micrograph of the liver section in resveratrol (20 mg/kg) group (c), normal liver structure. Micrograph of liver section in resveratrol + malathion (20 mg/kg) group (d), normal liver structure
Discussion
Organophosphorus compounds from among pesticides have the most toxicity for vertebrates.[4] One of the most effective organophosphorus compounds in the world is malathion and diazinon.[26] The toxicity of organophosphorus compounds can lead to blood and biochemical changes, oxidative stress, and lipid peroxidation. Therefore, another mechanism for toxicity to organophosphorus compounds is oxidative stress.[5] The findings revealed that, compared to the normal control group, the number of hepatocytes in the control group of malathion has significantly decreased, whereas the central venous size has significantly increased. In addition, there were a significant increase in the number of hepatocytes and a significant reduction in the size of the central venous in recipient groups resveratrol and malathion + resveratrol in all doses as compared to the control malathion group. Another important finding was that some changes in the liver were observed in the control group of malathion that was in the form of the plethoric state of the sinuses, macrophage accumulation around the central veins and the lymphoid cell penetration in the port space, as well as the central vein diameter enlargement. It seems that the invasion of free radicals to liver cells causes necrosis in parenchymal cells. These cells can induce inflammatory responses in the liver, which leads to tissue damage by single-nucleus inflammatory cells. The necrotic cells release the pro-inflammatory mediators and it can exacerbate poison-induced liver injury.[27] Apparently, macrophages are activated in response to tissue injury and release-positive mediators, such as the alpha-tumor necrosis factor, interleukin-1, and nitrite oxide.[11] In the present study, macrophages are actually the same as copper cells that are in the liver sinuses. It may seem that the copper cell accumulation and the secretion of toxic mediators in the areas, that have not undergone necrosis yet, are involved in causing liver toxicity and necrosis.[28] Moreover, free radicals' production and subsequent oxidative stress can be one of the most critical and essential causes of the liver cell death.[13] The results corroborate the ideas of Chakraborty et al. who suggested that the liver injury and apoptosis induction in hepatocytes can be caused by malathion.[29] Malathion induced of free radicals may invade to liver cells and cause necrosis in parenchymal cells. These cells can trigger inflammatory responses in the liver and cause the single-nucleus inflammatory cells to invade the tissue injury. The necrotic cells release the pro-inflammatory mediators, and this can exacerbate toxin-induced liver injury.[30] It may seem that the oxidative stress, which is induced by malathion administration, can induce the production of active oxygen species, and some notable examples are hydroperoxides, singlet oxygen, hydrogen peroxide, and superoxide that lead to the destruction of cell, DNA, proteins, and intracellular lipids and ultimately to liver injury.[31] Resveratrol appears to carry out a protective effect against fibrogenesis on the liver through polyphenol capacity and by inhibiting the stellate cell activity, destroying the transduction signals' way, and expressing the cell cycle protein.[18] Stellate cells play a crucial part in the improvement of liver fibrosis and oxidative stress.[11] It seems that resveratrol has the capability to inhibit P38MAPK phosphorylation in the activated LPS in microglia. Resveratrol can exert its anti-inflammatory effects on NF-Kβ. Resveratrol can inhibit NF-Kβ by reducing H2O2 production, inhibiting IKβ kinase and phosphorylation P65, and depleting P65.[17] Kasdallah-Grissa et al. illustrated that resveratrol inhibits the induction of ethanol's undesirable effects through the inhibition of lipid's induction, which is in line with the findings of the current study.[32] The results of this study indicate that there is a significant difference between liver antioxidant capacity and AST, ALT, and ALP levels in the control malathion group and the normal control group, respectively. Similarly, there is a negative correlation, in all doses, between antioxidant capacity of liver tissue in the control malathion group and AST, ALT, and ALP levels in the group that received resveratrol and malathion + resveratrol. The increase in the activity of liver function index enzymes in serum indicates a liver injury in the current study. These enzymes can be released into the bloodstream due to the incidence of necrosis or cell membrane damage.[11] It may seem that malathion can induce damage to the cell membrane integrity by the inhibition of complex I–IV and the respiratory chain.[33] The results are in agreement with Mehri et al. findings which revealed that the malathion administration in male rats for 1 week induces the increase of liver enzymes and conversely reduces the total antioxidant capacity.[34] Resveratrol appears to stabilize cell membranes and prevents leakage of enzymes by preventing lipid peroxidation.[13] Resveratrol can exert its antioxidant and anti-inflammatory effects by inducing antioxidant enzymes, adjusting lipid metabolism, and reducing lipid peroxidation.[20] The results are consistent with those of Schmatz et al. who suggested that administration of resveratrol reduces liver enzymes in diabetic rats and prevents injuries to hepatocytes.[35] The current study results also showed that resveratrol is able to reduce lipid peroxidation (decreased MDA) and increase antioxidant capacity (increased FRAP) of liver tissue, thus reducing oxidative stress. Consistent with these findings, a large body of studies has shown antioxidant properties for resveratrol.[161736] Thus, it appears that resveratrol with its antioxidant properties could reduce MDA and increase FRAPS in the treatment groups by inhibiting the production of ROS. The results of this study indicated a significant increase in the amount of nitrite oxide in serum of the recipient control malathion group compared to the normal control group. Furthermore, there was a significant decrease in the recipient malathion + resveratrol group in serum nitrite oxide level compared to the control malathion group. It seems that oxidative stress in cells increases the synthesis of nitrite oxide synthase and consequently leads to increase in nitrite production and decrease in cell survival.[37] Due to the high consumption of oxygen, mitochondrial dysfunction may increase the production of free radicals in most tissues of the body, including nitrite oxide radicals, and due to the oxidative and nitrosative stress, it may induce injury to the tissues, especially the liver.[38] Administration of malathion through the induction of oxidative stress can significantly increase the amount of nitrotyrosine and NO biomarkers in the liver.[39] On the other hand, antioxidants can damage and degrade the NO system (protein enzymes, substrates, and cofactors) and hence reduce NO production.[20] The results are in agreement with of Jalili et al. findings which showed that resveratrol can reduce nitrite oxide in morphine-induced damage to the kidney.[14] The results are in accord with recent studies, indicating that malathion, one of the organophosphorus compounds, is apparently able to damage and degrade hepatocytes, reduce antioxidant capacity, and elevate serum levels of liver enzymes and nitrite oxides by inducing oxidative stress. On the contrary, resveratrol, a potent antioxidant agent, can reduce the destructive effects of these organophosphates to some extent. Accordingly, using food and fruits that are high in resveratrol can be a good strategy to reduce free radicals and prevent injuries to the liver of the people who are exposed to organophosphorus compounds, specifically pesticides.
Conclusion
It appears that resveratrol provides protection against oxidative stress resulting from malathion in the liver and such an ability of the resveratrol might be due to its strong potential antioxidant attributes. Resveratrol administration moderates the detoxification enzymes, and the antioxidant agents in the extract bring about hepatic tissue recovery and prevention of malathion adverse effects on NO, ALT, AST, and ALP as evidenced in the abovementioned examination of the male Wistar rats.
Financial support and sponsorship
Nil.
Conflicts of interest
There are no conflicts of interest.
Acknowledgments
This work was conducted in partial fulfillment of the requirements of MD degree which was financially (Grant No. 96612) supported by the Research Council of Kermanshah University of Medical Sciences, Kermanshah, I. R. Iran.
References
- Biocatalytic membrane reactor development for organophosphates degradation. J Hazard Mater. 2019;365:789-95.
- [Google Scholar]
- Biphilic organophosphorus-catalyzed intramolecular Csp2-H amination: Evidence for a nitrenoid in catalytic cadogan cyclizations. J Am Chem Soc. 2018;140:3103-13.
- [Google Scholar]
- Trends and perspectives in immunosensors for determination of currently-used pesticides: The case of glyphosate, organophosphates, and neonicotinoids. Biosensors (Basel). 2019;9 pii: E20
- [Google Scholar]
- Significance of serum cholinesterase level in organophosphate poisoning. Med Leg Update. 2012;1:70-4.
- [Google Scholar]
- Oxidative stress after acute and sub-chronic malathion intoxication in wistar rats. Environ Toxicol Pharmacol. 2007;23:198-204.
- [Google Scholar]
- Assessment of thymoquinone effects on apoptotic and oxidative damage induced by morphine in mice heart. Cell Mol Biol (Noisy-le-grand). 2018;64:33-8.
- [Google Scholar]
- Toxicity of malathion at early life stages of the Senegalese sole, Solea senegalensis (Kaup, 1858): Notochord and somatic disruptions. Histol Histopathol. 2018;33:157-69.
- [Google Scholar]
- A refined ecological risk assessment for California red-legged frog, delta smelt, and California tiger salamander exposed to malathion. Integr Environ Assess Manag. 2018;14:224-39.
- [Google Scholar]
- Malathion-induced oxidative stress in rat brain regions. Neurochem Res. 2006;31:671-8.
- [Google Scholar]
- In vitro evaluation of selenium genotoxic, cytotoxic, and protective effects: A review. Arch Toxicol. 2010;84:337-51.
- [Google Scholar]
- Genistein improves liver damage in male mice exposed to morphine. Chin Med J (Engl). 2018;131:1598-604.
- [Google Scholar]
- Cardiac oxidative stress determination and myocardial morphology after a single ecstasy (MDMA) administration in a rat model. Int J Legal Med. 2008;122:461-9.
- [Google Scholar]
- The effects of thymoquinone against morphine-induced damages on male mice liver. Int J Prev Med. 2018;9:8.
- [Google Scholar]
- Protective effect of resveratrol against morphine damage to kidneys of mice. Int J Morphol. 2017;35:1409-15.
- [Google Scholar]
- Growth inhibitory and antimetastatic effect of green tea polyphenols on metastasis-specific mouse mammary carcinoma 4T1 cells in vitro and in vivo systems. Clin Cancer Res. 2005;11:1918-27.
- [Google Scholar]
- Multiple molecular targets of resveratrol: Anti-carcinogenic mechanisms. Arch Biochem Biophys. 2009;486:95-102.
- [Google Scholar]
- Resveratrol inhibits nitric oxide and TNF-alpha production by lipopolysaccharide-activated microglia. Int Immunopharmacol. 2005;5:185-93.
- [Google Scholar]
- Resveratrol induces nuclear factor-κB activity in human cardiac cells. Int J Cardiol. 2013;167:2507-16.
- [Google Scholar]
- Therapeutic effect of resveratrol on morphine-induced damage in male reproductive system of mice by reducing nitric oxide serum level. Int J Morphol. 2017;35:1342-7.
- [Google Scholar]
- High absorption but very low bioavailability of oral resveratrol in humans. Drug Metab Dispos. 2004;32:1377-82.
- [Google Scholar]
- Resveratrol inhibits cyclooxygenase-2 transcription and activity in phorbol ester-treated human mammary epithelial cells. J Biol Chem. 1998;273:21875-82.
- [Google Scholar]
- Potential of resveratrol in anticancer and anti-inflammatory therapy. Nutr Rev. 2008;66:445-54.
- [Google Scholar]
- Involvement of brain-derived neurotrophic factor (BDNF) on malathion induced depressive-like behavior in subacute exposure and protective effects of crocin. Iran J Basic Med Sci. 2015;18:958-66.
- [Google Scholar]
- Therapeutic effects of curcumin on renal tissue damages induced by ischemia reperfusion in rat. Koomesh. 2015;16:273-81.
- [Google Scholar]
- Sub-acute effects of diazinon on biochemical indices and specific biomarkers in rats: Protective effects of crocin and safranal. Food Chem Toxicol. 2010;48:2803-8.
- [Google Scholar]
- Behavioral, neurochemical, and neuromorphological effects of deltamethrin in adult rats. J Toxicol Environ Health. 1996;48:515-26.
- [Google Scholar]
- Protective effect of edible turmeric powder on early hepatic injury in diabetic rats. J Kashan Univ Med Sci. 2010;14:190-9.
- [Google Scholar]
- Studies on L-ascorbic acid metabolism in rats under chronic toxicity due to organophosphorus insecticides: Effects of supplementation of L-ascorbic acid in high doses. J Nutr. 1978;108:973-80.
- [Google Scholar]
- An in vivo study in mice: Mother's gestational exposure to organophosphorus pesticide retards the division and migration process of neural progenitors in the fetal developing brain. Toxicol Res (Camb). 2016;5:1359-70.
- [Google Scholar]
- Investigation of the toxicity of some organophosphorus pesticides in a repeated dose study in rats. Rom J Morphol Embryol. 2013;54:349-56.
- [Google Scholar]
- Protective effect of resveratrol on ethanol-induced lipid peroxidation in rats. Alcohol Alcohol. 2006;41:236-9.
- [Google Scholar]
- Prevention of malathion-induced depletion of cardiac cells mitochondrial energy and free radical damage by a magnetic magnesium-carrying nanoparticle. Toxicol Mech Methods. 2010;20:538-43.
- [Google Scholar]
- Hepatoprotective effect of the root extract of green tea against malathion-induced oxidative stress in rats. J HerbMed Pharmacol. 2016;5:116-9.
- [Google Scholar]
- Effects of resveratrol on biomarkers of oxidative stress and on the activity of delta aminolevulinic acid dehydratase in liver and kidney of streptozotocin-induced diabetic rats. Biochimie. 2012;94:374-83.
- [Google Scholar]
- The subchronic exposure to malathion, an organophosphate pesticide, causes lipid peroxidation, oxidative stress, and tissue damage in rats: The protective role of resveratrol. Toxicol Res (Camb). 2018;7:503-12.
- [Google Scholar]
- Resveratrol protects ROS-induced cell death by activating AMPK in H9c2 cardiac muscle cells. Genes Nutr. 2008;2:323-6.
- [Google Scholar]
- Antisense oligodeoxynucleotides to inducible NO synthase rescue epithelial cells from oxidative stress injury. Am J Physiol. 1996;270:F971-7.
- [Google Scholar]
- Oxidative stress and alteration of biochemical markers in liver and kidney by malathion in rat pups. Toxicol Ind Health. 2015;31:783-8.
- [Google Scholar]