Translate this page into:
Hemoglobin Oxidation in Stored Blood Accelerates Hemolysis and Oxidative Injury to Red Blood Cells
Address for correspondence Ibrahim Mustafa, PhD, Department of Biomedical Science, College of Health Science, QU- Health, Qatar University, P.O. Box 2713, Doha, Qatar (e-mail: ibrahim.mustafa@qu.edu.qa).
This article was originally published by Thieme Medical and Scientific Publishers Pvt. Ltd. and was migrated to Scientific Scholar after the change of Publisher.
Abstract
Introduction
Maintaining blood supply is a challenge in blood banks. Red blood cells (RBCs) stored at 4°C experience issues of biochemical changes due to metabolism of cells, leading to changes collectively referred to as “storage lesions.” Oxidation of the red cell membrane, leading to lysis, contributes to these storage lesions.
Methods
Blood bags with CPD-SAGM stored at 4°C for 28 days were withdrawn aseptically on days 1, 14, and 28. Hematology analyzer was used to investigate RBC indices. Hemoglobin oxidation was studied through spectrophotometric scan of spectral change. RBC lysis was studied with the help of Drabkin's assay, and morphological changes were observed by light and scan electron microscopy.
Results
RBCs show progressive changes in morphology echinocytes and spherocytes on day 28. There was 0.85% RBC lysis, an approximately 20% decrease in percentage oxyhemoglobin, and a 14% increase in methemoglobin formation, which shows hemoglobin oxidation on day 28.
Conclusions
Oxidative damage to RBC, with an increase in storage time was observed in the present study. The observed morphological changes to RBC during the course of increased time shows that there is progressive damage to RBC membrane and a decrease in hemoglobin concentration; percentage RBC lysis is probably due to free hemoglobin and iron.
Keywords
storage lesions
erythrocytes
free hemoglobin
iron
Introduction
Great efforts have been made to provide a suitable and safe supply of blood with more benefits than side effects. Currently, the storage procedures of blood bags in blood banks require some conditions to ensure the maximum storage time for a healthy and safe blood supply. Nowadays, blood bags can be stored up to 42 days at 2 to 6°C, as long as the mean hemolysis does not exceed 0.8% and more than 75% red blood cells (RBCs) survive in the first 24 hours posttransfusion.1 The past two decades have witnessed increased scrutiny regarding efficacy and risk of the once unquestioned therapy of RBC transfusion. Simultaneously, a variety of changes have been identified within the RBC and storage media during RBC preservation, which are correlated with reduced tissue oxygenation and transfusion-associated adverse effects.2 These alterations are collectively termed the storage lesions and include extensive biochemical, biomechanical, and immunologic changes involving cells of diverse origin.3
Blood transfusions save lives, and yet millions of patients requiring transfusions do not have timely access to safe blood. It is important to provide safe blood that is free from transfusion-transmissible diseases and also reduce the risk of the adverse effects of blood transfusions. However, there is a risk of injury due to transfusion of stored old blood, such as transfusion-associated acute lung injury (TRALI).4 There is a risk of mortality and morbidity in critically ill patients who receive stored old blood.5,6
Storage of RBCs in a preservative medium is associated with harmful metabolic, biochemical and molecular changes to erythrocytes: these changes are collectively referred to as “storage lesions.”7 Blood products such as RBCs stored with additive solutions in different temperatures contribute to storage lesions significantly.8 The most probable sites of damage will be cytoskeletal membrane proteins in RBC. These membrane changes will make RBC fragile and increase osmotic fragility, biochemical changes and electrolyte imbalance.9 When stored blood ages intraerythrocytically, metabolic activities are reduced due to the depletion of energy sources, leading to instability in cytoskeletal membrane. Because of this, cells are very fragile as they age, and deformability is observed. Because of osmotic fragility, cell-free hemoglobin and microparticles are released. When stored blood is transfused, cell-free hemoglobin and microparticles lead to further hemolysis and breakdown of RBC.10
Understanding the oxidative damage to blood products and biological markers to oxidative stress may help in developing inhibitory mechanisms to those factors leading to oxidative stress for RBCs. One of the leading causes of oxidative damage may be iron-mediated reactive oxygen species that indiscriminately cause damage to major macromolecules such as protein and lipids on cells.11 In stored blood, due to hemolysis, free hemoglobin rich in iron may cause progressive oxidative stress to stored RBC. There are studies that show ferric iron accelerates hemoglobin-mediated oxidation, which can be inhibited by iron-chelating agents.12 We believe biocompatible iron chelators may be used in blood products such as platelets and other cellular products in blood banks. If biocompatible iron chelators can be utilized in additive solutions, this may have commercial benefits to produce, for example, platelet bags to improve shelf life, which may benefit the health budgets of developing countries; also, it may have benefits for patients globally. The development of biocompatible iron chelators will be a cheaper and cost-effective method to make these additive solutions in platelets.
The aim of the present study was to investigate oxidative injury to RBCs during storage for 28 days in standard storage temperature of 4 to 6°C by measuring % oxyhemoglobin, % methemoglobin due to hemoglobin oxidation, RBC lysis due to hemolysis, and the correlation of oxidative injury with morphological changes during the course of storage time.
Materials and Methods
The blood bags were obtained from Hamad Medical Cooperation (HMC) blood donor center at Doha, Qatar. Institutional review board (IRB) approval was obtained from HMC for using the donor’s blood for the purpose of research. Whole blood, 450 mL, was collected from healthy volunteer donors in CPD-SAGM, according to standard blood bank procedures. The blood units were stored at 4 to 6°C for 28 days and samples were withdrawn aseptically on day 0, day 14, and day 28 for the investigations. Some of the samples collected from the blood bags were utilized for hematological analysis using Sysmex XE5000.
RBC Morphology
To demonstrate RBC storage lesions due to oxidative damage, it is important to see those abnormalities morphologically. To see the RBCs morphologically, Romanoskey stained wedge smears made from the blood collected from the blood bags at different time points. To see the morphological changes more clearly, we have used scanning electron microscopy on fixed cells with glutaraldehyde.
Light Microscopy
Blood smears on a glass microscope slide were made by adding a small drop of blood on the surface and on one end of the slide; thereafter, by using the edge of another clean slide, the drop was pulled over the whole surface of the slide by the wedge technique. The slide was air-dried and coated on one side with a thin layer of whole blood, stained with the Wright's stain for 5 minutes, washed with running water, cleaned, dried, and examined under the light microscope OLYMPUS BX41 on oil immersion with 100x objective.
Scan Electron Microscopy
Blood samples were fixed in phosphate-buffered saline with 3% glutaraldehyde for 2 days at 4°C, and then the samples were washed three times in saline buffer and centrifuged to separate the blood from the buffer and glutaraldehyde. Then, samples dehydrated in an ascending graded alcohol series (50, 60, 70, 80, 90 and 100%) were fixed in ethyl alcohol. Each time the samples were left with the graded alcohol for 15 minutes at 4°C incubator, following which they were left for 30 minutes in absolute alcohol and repeated for three times. Finally, the samples were sent in 100% ethyl alcohol to the electron microscopic examination in the central laboratory of Qatar University. Therein, the technician left the sample for air-drying for around 2 days and then covered them with a layer of gold palladium before undergoing scanning electron microscopy analysis. The type of electron microscope used was the reflecting electron microscope.
Hemoglobin-mediated Investigations
RBC Lysis
Total hemoglobin and percentage RBC lysis were determined using Drabkin’s assay. Briefly, Drabkin’s reagent converted hemoglobin to cyanomethemoglobin, which absorbs light at 540 nm. Aliquots whole blood were removed from blood bags, and immediately 40 µL of blood was mixed with Drabkin’s reagent to determine the total hemoglobin concentration.13 The remaining cell suspension in the aliquot of each sample was centrifuged, and the supernatant was removed and mixed with Drabkin’s reagent. Both total and supernatant hemoglobin concentrations were calculated from the OD at 540 nm as grams of hemoglobin per 100 mL of solution (g%):
Subsequently, the supernatant hemoglobin was calculated as a percentage of total hemoglobin to give percentage RBC lysis in the following manner:
The percentage of RBC lysis was determined by using 1 mL of Drabkin’s reagent that was mixed with 40 µL of the plasma, which was separated from the blood by centrifugation at 3000 rpm for 5 minutes. The function of Drabkin’s reagent is to convert hemoglobin to cyanomethemoglobin, which absorbs light at 540 nm. Then, the samples were examined for the absorbance in the SPEKOL 1300 UV-VIS Spectrophotometer. Another part of RBC lysis test involved determination of hemoglobin concentration in whole blood, which was performed similarly as the Drabkin’s assay.
Hemoglobin Oxidation
Hemolysates were prepared by hypotonic lysis of pRBC in H2O and were adjusted to a final concentration of approximately 40 µM heme at a final pH of between 7.1 to 7.4. Hemoglobin oxidation in hemolysate was quantified by spectrophotometric analysis (500–700 nm).14 The scans were done using a fixed method selected from the dual beam spectrophotometer, with a cycle time of 31 seconds for 10 cycles. The concentrations of oxyhemoglobin, methemoglobin, and hemichrome were calculated from the absorption spectra, according to the method of Winterbourn.12,15 The calculations were made using µM extinction coefficients as described in the method.
Statistical Analysis
All results are expressed as mean ± standard error mean (SEM). The results are expressed as the mean (per day) ± SEM. A minimum of three replicates was performed for all studies, although in some cases (e.g., microscopy and spectral scan) representative data were presented.
Results
Hemoglobin Oxidation
As demonstrated here in ►Fig. 1, there is no significant change in spectra to show hemoglobin oxidation at day 1. Surprisingly, it showed a < 15% decrease in oxyhemoglobin concentration within 28 days of storage time, which is quite significant compared with day 1. To correlate hemoglobin oxidation due to free iron, an experiment was performed by making a hemolysate, which was challenged with 250µM F3+ and Fe2+. The results showed that within 30 seconds, that there was 250 mM Fe3+ added sample spectral change, where due to methemoglobin formation, normal spectral peaks were lost, whereas there was no change in Fe2+ added sample (►Fig. 2)

- The data shown is spectra of hemolysate scan, calculated % oxyhemoglobin, and % methemoglobin derived from the spectral change. The graph A shows at day 1, the spectra with 99% oxyhemoglobin, while B shows slight changes in the spectra at 630 nm, indicating methemoglobin. Graph C and D shows % oxyhemoglobin and % methemoglobin during the storage time. The values shown are the mean ± SD of three independent experiments.
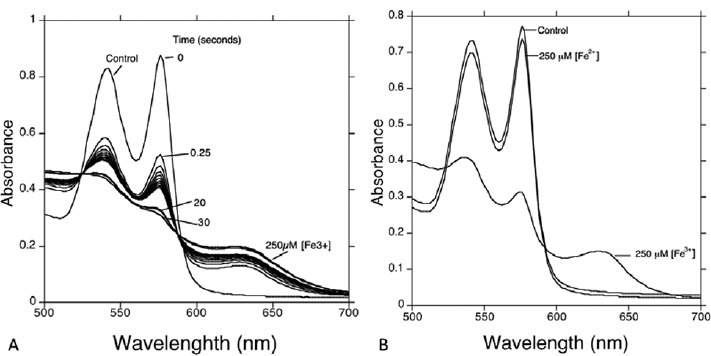
- Absorption spectra of Control oxyHb, 250 µM Fe3+ in plate A. In plate B, 250 µM Fe3+. Control oxyHb, 250 µM Fe2+, and 250 µM Fe3+. All solutions are approximately 40 µM HbA hemolysate in phosphate buffer (pH 7.4).
Microscopy
Further investigations were done with an advanced electron microscope to see the morphology of RBC changes. For light microscopy, Wright stained wedge smears were observed by a light microscope with oil immersion; on day 1, the cells appeared normocytic normochromic, but by days 14 to 28, approximately 50% of the cells showed crenation, and spherocytes were observed in ►Fig. 3.

- Blood smear stained with Wright’s stain for light microscopy of RBCs from a blood bag collected in CPD-SAGM. The micrograph with magnification 100x shows cells are morphologically normal at (A) day 1, (B) day 14, and (C) day 28. The majority of cells show crenation and spherocytes. A1 and C1 are magnified to show days 1 and 28. Samples were taken directly from blood bags, wedge smears were made immediately, and air-dried slides were stained.
As shown in ►Fig. 4, scan electron microscopy shows the RBCs were normal with discoid, biconcave shape on day 1. However, at day 28, higher magnification SEM showed progressive changes in the morphology of cells, with lots of cells showing crenate thorns on the surface of RBCs, and some cells becoming echinocytes and spherocytosis in ►Fig. 5.
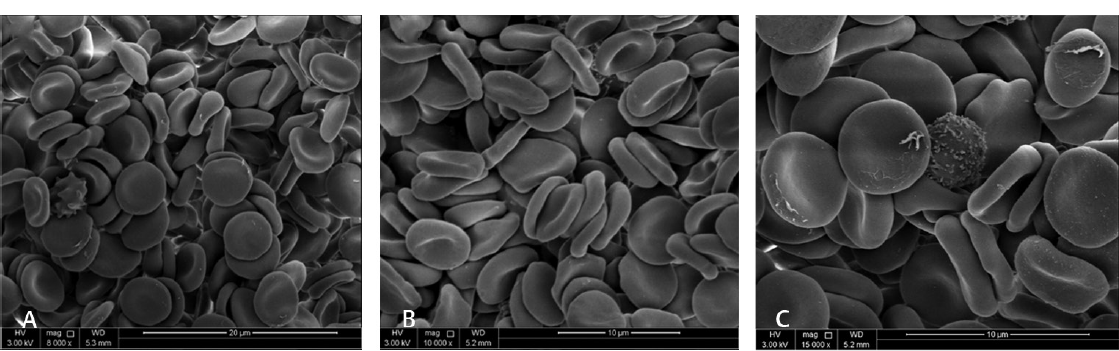
- Low- and high-magnification of scan electron microscopy of RBCs from a blood bag on day 1 of blood donation collected in CPD-SAGM. The micrograph A-C with magnification 8,000× to 15,000×, respectively, shows cells are morphologically normal. Samples were prepared with packed RBC in buffered saline and fixed with 3% gulutraldehyde.

- Low- and high-magnification of scan electron microscopy of RBCs from a blood bag on day 28 of blood donation collected in CPD-SAGM. The micrograph A-C with magnification 8,000× to 15,000×, respectively, shows cells are morphologically changing to echinocytes and spherocytes. Samples were prepared with packed RBC in buffered saline and fixed with 3% gulutraldehyde.
Discussion
RBCs have a lifespan of 120 days in vivo, and senescence of these delicate cells are associated with many functions of biochemical and biophysical series of events, similar to stored blood.16 It is important to know the bulk of iron in the human body is located within the erythroid series of cells and mature RBCs.17 The normal RBC is the most ferruginous somatic cell, containing approximately 20 mM iron.14 Indeed, approximately 55% of the iron in the human body is in the form of hemoglobin and myoglobin.18 Free hemoglobin has the ability to catalyze to form powerful oxidants such as hydroxyl radical via Fenton-type reactions, where heme Fe2+ decomposes by H2O2 to form methemoglobin.19
In our study, we have demonstrated in ►Figs. 1 and 6, that there was 0.85% RBC lysis, approximately 20% decrease in percentage oxyhemoglobin, and a 14% increase in methemoglobin formation, which shows hemoglobin oxidation on day 28 compared with day 1. In agreement with other studies,9,20 we have also demonstrated hemolysis during the span of increased storage time to 28 days. It is not a common practice to check blood products for their quality of biophysical and biochemical aspects as a form of pretransfusion testing for therapeutic usage in clinical practice. Perhaps, it may be useful to use simple hemoglobin scan spectral change to monitor the amount of methemoglobin in stored blood before transfusion, as we have demonstrated. There are studies that show the increase in the negative effects of the transfusion of RBC in proportion to the duration of storage of the blood product in specific patients.21
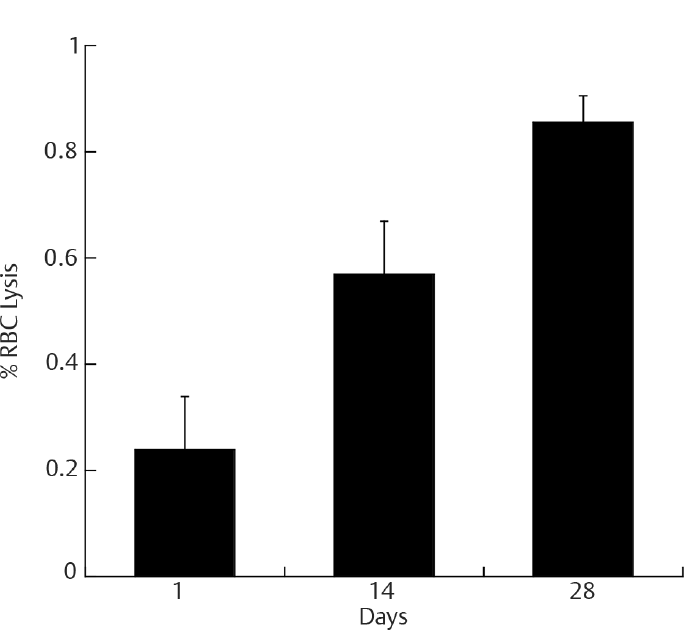
- RBC hemolysis with an increase in storage time. The data shown is RBCs taken from blood bag with whole blood in CPD-SAGM stored at 4°C for 28 days. At day 1, there is no significant RBC lysis, but as storage time increased, there was significant RBC lysis. The data are triplicate samples analyzed at each time point. The values shown are the mean ± SD of three independent experiments.
With regard to the RBC morphology, the normal morphology was lost during the storage. As it was shown, the morphology of the RBC on day 1 was normal with discoid and biconcave shape, while between days 14 to 28, the cells showed progressive morphological changes of crenation, and cells were seen as echinocytes and spherocytes.
Many clinical studies have been done to identify the relationship between the duration of RBC storage, changes that occurred at the molecular level, and the side effects in the patients who required blood transfusion to determine to what extent RBCs stored for a long period lose safety and efficacy.22,23
There are many side effects seen in some critically ill patients after multiple transfusions of old RBCs showed oxygen uptake with sepsis patients does not record beneficial effect.22 Transfusions with stored old blood increase the rate of mortality.24 Transfusion of old blood has also caused multiorgan failure, increased susceptibility to infections,25,26 and led to development of complications such as transfusion-related acute lung injury (TRALI).27
Lipid peroxidation of RBC membrane, as well as RBC cytoskeletal protein changes due to oxidative damage and its numerous functional implications, are reported in several studies.28 As we believe the oxidative damage shown in the present work may be due to the free iron from hemoglobin, plasma iron and RBC iron will be assessed by ICP mass spectrophotometry. Further studies will be done to see how far this oxidative damage can be inhibited with respect to iron by using biocompatible iron chelating agents as additive solutions to blood bags.
Conclusion
Oxidative damage to RBC with an increase in storage time was observed in the present study. To correlate the oxidative damage, further investigation of metabolic and biochemical investigation is required. The observed morphological changes in RBCs during the course of increased time made it obvious that there is progressive damage to RBC membrane and decrease in hemoglobin concentration, and percentage RBC lysis is probably due to free hemoglobin and iron; therefore, this oxidative damage can be minimized by antioxidants such as biocompatible iron chelators.
Acknowledgment
The authors are grateful to Dr. Zeyd Merenkov from the Blood Bank of Hamad Medical Cooperation and Asma Al Marwani from the Hematology Department for assisting in this research. The authors also appreciate the efforts of Areej Awadallah, Muna Mohammed Al-Kafaween from Qatar University Biomedical Science, who helped in collecting some data and Mr. Essam Attia at the Qatar University Central Laboratory for assisting with the electron microscopy. The research is supported by Qatar University student grants.
Conflicts of Interest
None.
Reference
- Red blood cell storage lesion. Bosn J Basic Med Sci. 2009;9(Suppl. 01):21-27.
- [CrossRef] [PubMed] [Google Scholar]
- Bad blood: the risks of red cell storage. Nat Med. 2010;16(04):381-382.
- [CrossRef] [PubMed] [Google Scholar]
- Age of red blood cells and transfusion in critically ill patients. Ann Intensive Care. 2013;3(01):2.
- [CrossRef] [PubMed] [Google Scholar]
- Effect of red blood cell transfusion on parameters of inflammation and oxidative stress in critically ill patients. Rev Bras Ter Intensiva. 2011;23(01):30-35.
- [CrossRef] [PubMed] [Google Scholar]
- Oxidative injury as contributory factor for red cells storage lesion during twenty eight days of storage. Blood Transfus. 2012;10(01):59-62.
- [Google Scholar]
- Time to revisit red blood cell additive solutions and storage conditions: a role for “omics” analyses. Blood Transfus. 2012;10(Suppl. 02):s7-s11.
- [Google Scholar]
- Time dependent assessment of morphological changes: leukodepleted packed red blood cells stored in SAGM. BioMed Res Int. 2016;2016 4529434
- [CrossRef] [PubMed] [Google Scholar]
- Storage lesion: role of red blood cell breakdown. Transfusion. 2011;51(04):844-851.
- [CrossRef] [PubMed] [Google Scholar]
- Biological markers of oxidative stress: Applications to cardiovascular research and practice. Redox Biol. 2013;1(01):483-491.
- [CrossRef] [PubMed] [Google Scholar]
- In vitro chelating, cytotoxicity, and blood compatibility of degradable poly(ethylene glycol)-based macromolecular iron chelators. Biomaterials. 2009;30(04):638-648.
- [CrossRef] [PubMed] [Google Scholar]
- Photolysis and membrane lipid peroxidation of human erythrocytes by m-chloroperbenzoic acid. Clin Chim Acta. 1998;277(01):1-11.
- [CrossRef] [PubMed] [Google Scholar]
- Thalassaemic erythrocytes: cellular suicide arising from iron and glutathione-dependent oxidation reactions? Br J Haematol. 1995;91(04):811-819.
- [CrossRef] [PubMed] [Google Scholar]
- Oxidative reactions of hemoglobin. Methods Enzymol. 1990;186:265-272.
- [CrossRef] [PubMed] [Google Scholar]
- Biophysical and biochemical markers of red blood cell fragility. Transfus Med Hemother. 2017;44(03):183-187.
- [CrossRef] [PubMed] [Google Scholar]
- An update on iron physiology. World J Gastroenterol. 2009;15(37):4617-4626.
- [CrossRef] [PubMed] [Google Scholar]
- Review on iron and its importance for human health. J Res Med Sci. 2014;19(02):164-174.
- [Google Scholar]
- Hemoglobin. A biologic Fenton reagent. J Biol Chem. 1984;259(23):14354-14356.
- [CrossRef] [PubMed] [Google Scholar]
- Red blood cell storage and cell morphology. Transfus Med. 2012;22(02):90-96.
- [CrossRef] [PubMed] [Google Scholar]
- Microvascular response to red blood cell transfusion in patients with severe sepsis. Crit Care Med. 2007;35(07):1639-1644.
- [CrossRef] [PubMed] [Google Scholar]
- Effect of stored-blood transfusion on oxygen delivery in patients with sepsis. JAMA. 1993;269(23):3024-3029.
- [CrossRef] [PubMed] [Google Scholar]
- Age of transfused blood: an independent predictor of mortality despite universal leukoreduction. J Trauma. 2008;65(02):279-282. discussion 282–284
- [CrossRef] [PubMed] [Google Scholar]
- Association of mortality with age of blood transfused in septic ICU patients. Can J Anaesth. 1997;44(12):1256-1261.
- [CrossRef] [PubMed] [Google Scholar]
- Age of transfused blood is an independent risk factor for postinjury multiple organ failure. Am J Surg. 1999;178(06):570-572.
- [CrossRef] [PubMed] [Google Scholar]
- Increased rate of infection associated with transfusion of old blood after severe injury. Arch Surg. 2002;137(06):711-716. discussion 716–717
- [CrossRef] [PubMed] [Google Scholar]
- Transfusion-related acute lung injury. Blood. 2005;105(06):2266-2273.
- [CrossRef] [PubMed] [Google Scholar]
- Duration of red blood cell storage and inflammatory marker generation. Blood Transfus. 2017;15(02):145-152.
- [Google Scholar]